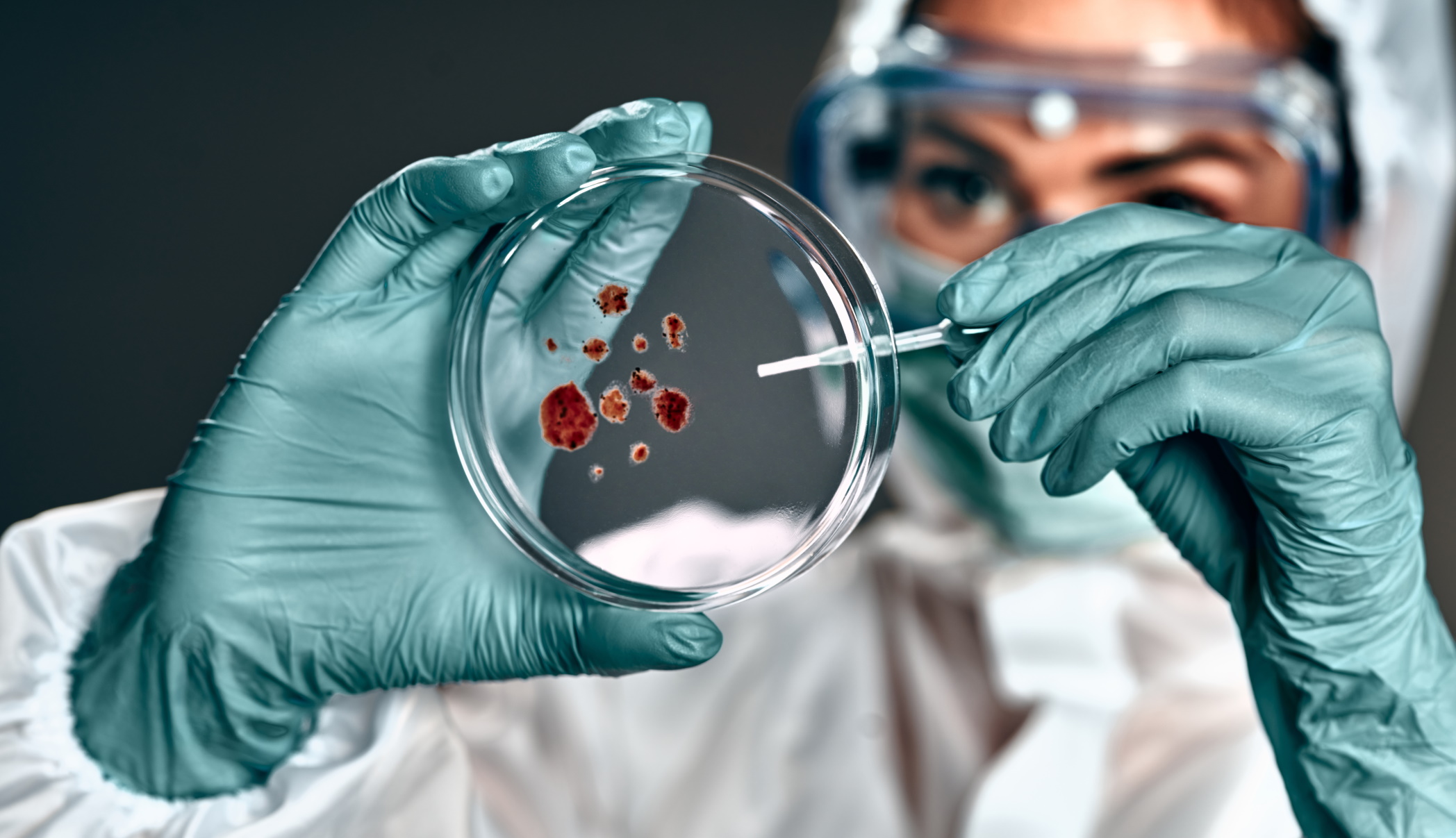
Challenges in Biomaterials’ Clinical Translation: Navigating Innovation and Complexity
Over the years, biomaterials have evolved significantly, driven by constant innovation and advancements in clinical medicine. Initially defined as nonviable materials intended to interact with biological systems, biomaterials now encompass a diverse range of classes including natural materials, polymers, metals, bioceramics, and composites. The boundaries between these classes have become increasingly blurred, due to combined technology that harnesses the unique properties of different materials to create superior innovations. To achieve successful clinical translation, researchers must have a comprehensive understanding of the product development process, considering the end-goal and biological complexities of physiological and pathological environments. Automated manufacturing processes and standardised fabrication methods play a crucial role in scaling up production, while assessing commercial viability and navigating regulatory approval are vital for protecting intellectual property and securing necessary investments. Despite the obstacles, collaboration between clinicians and scientists holds the key to overcoming challenges and revolutionising patient care across various clinical disciplines through the power of biomaterial science.
Biomaterials play an integral role in today’s medicine, restoring function and facilitating the treatment of people after injuries or diseases. Biomaterials can be natural or synthetic and are used in medicine to support, reinforce, or replace damaged tissues or biological functions.
The first historical use of biomaterials dates back to ancient times. Ancient Egyptians used sutures made from animal tendons. The modern field of biomaterials combines medicine, biology, physics, and chemistry, as well as the latest influences from tissue engineering and materials science. In the last decade, this field has significantly advanced due to discoveries in tissue engineering, regenerative medicine, and many others.
Metals, ceramics, plastics, glass, and even living cells and tissues can be used to create biomaterials. They can be processed into moulded or machined parts, coatings, fibres, films, foams, and fabrics for use in biomedical products and devices. They can include heart valves, hip joint replacements, dental implants, or contact lenses. They often undergo biodegradation, and some are bio-absorbable, meaning they are gradually eliminated from the body after fulfilling their intended function [1].
The practical application of biomaterials in medicine presents many interesting challenges that scientists face. The goal is always the same – to ensure that the final product is durable and properly fulfils its functions. This certainly motivates intensive research efforts. Scientists bear a great responsibility because biomaterials are used, for example, in the creation of implants that need to perform specific functions for many years and cannot be constructed from compounds harmful to the human body. Figure 1 presents the pathway of invention of new material from the scientific idea to final product commercialisation.

Figure 1. The process of introducing a biomaterial to the market
Concept
The initial phase of developing a novel biomaterial involves identifying a specific need within a treatment modality or recognising gaps in the current literature. The creativity and expertise of researchers play a crucial role in generating innovative ideas, with collaborations between clinicians and scientists fostering discussions to enhance patient care.
The development of biomaterials for biomedical applications, such as artificial organs or implants, poses complex challenges for design engineers to meet market demands. Choosing a suitable material for a specific biomedical application requires considering its medical, technological, and economical aspects, including mechanical, physical, chemical, biological, and economical properties. Decision-makers must have a comprehensive understanding of product requirements and selection criteria to make informed decisions. The material selection problems can be effectively addressed by utilising multi-criteria decision (MCD) making methods, which offer a solution that considers numerous criteria, even when they are conflicting or difficult to compare [2].
Laboratory studies
Laboratory studies play a crucial role in the development of biomaterials. These studies involve in-depth characterisation and investigation of the interactions between the material and living tissues. However, it is necessary to emphasize the need to tailor these studies to specific tissues encountered by biomaterials, as there is no one-size-fits-all approach. It should be also noted that preliminary in vitro studies should be interpreted with caution, as they often fail to accurately simulate the complexity of tissues. Reproducing the mechanical environment within the extracellular matrix poses additional challenges, despite advancements in synthetic analogues and stem cell research. Consequently, prudent interpretation of in vitro results, particularly for studies lacking acceptable simulation of the tissue and environment, is recommended.
Business model
The business model could be categorised into five key aspects: patenting, registration, clinical trials, manufacture, and scale-up production. Cost emerges is a crucial factor that must be carefully considered alongside other elements of the model. One of the most important issues is the challenging nature of clinical translation, requiring substantial monetary investment. In order to be legally marketed, the biomaterial must obtain regulatory approval. This involves submitting comprehensive data and documentation to regulatory authorities, such as the FDA (Food and Drug Administration) in the United States or the EMA (European Medicines Agency) in Europe, for evaluation and approval [3]. The biomaterial undergoes rigorous clinical trials involving human participants. These trials are conducted in different phases to assess its safety, effectiveness, and potential side effects. Data collected during these trials is analysed and submitted for review. The hurdles associated with manufacturing and scaling up emphasize the need for consistent and reproducible techniques to optimise processes and increase production volumes while reducing costs. The sales volume of inventions hinges on the production cost and resulting consumer price, which should fall within an acceptable range to garner market interest and ultimately benefit patients.
Clinical applications
Reaching clinical entry for a material or device is a significant milestone, backed by substantial investments and hopes for market success. However, gaining buy-in from end users, particularly clinicians, remains a challenge due to their resistance to change and reliance on independent verification of claims. Independent research by university faculty helps confirm or refute product claims, focusing on long-term studies, post-operative sensitivity, retention of restorations, and marginal sealing ability. Clinical studies, cost-effectiveness, and outperforming existing alternatives are crucial for acceptance by clinicians. Collaborating with influential clinicians can drive acceptability and integration of novel materials or devices into clinical treatments.
Note: This article is based on Patel, E. (2020). Dental biomaterials: challenges in the translation from lab to patient. South African Dental Journal 75(1) pp. 16-28
Author: Agnieszka Piegat, Mirosława El Fray
References
[1] Vallet-Regí, M. (2022). Evolution of Biomaterials. Frontiers in Materials 9 (March), article 864016.
[2] Jahan, A. (2013). Weighting of dependent and target-based criteria for optimal decision-making in materials selection process: Biomedical applications. Materials and Design 49, pp. 1000-1008.
[3] Schuh, J.C.L. (2019). Compilation of International Standards and Regulatory Guidance Documents for Evaluation of Biomaterials, Medical Devices, and 3-D Printed and Regenerative Medicine Products. Toxicologic Pathology 47(3), pp. 344-357
Keywords
biomaterials, market, laboratory studies, business model, clinical applications, clinical translation, commercialisation