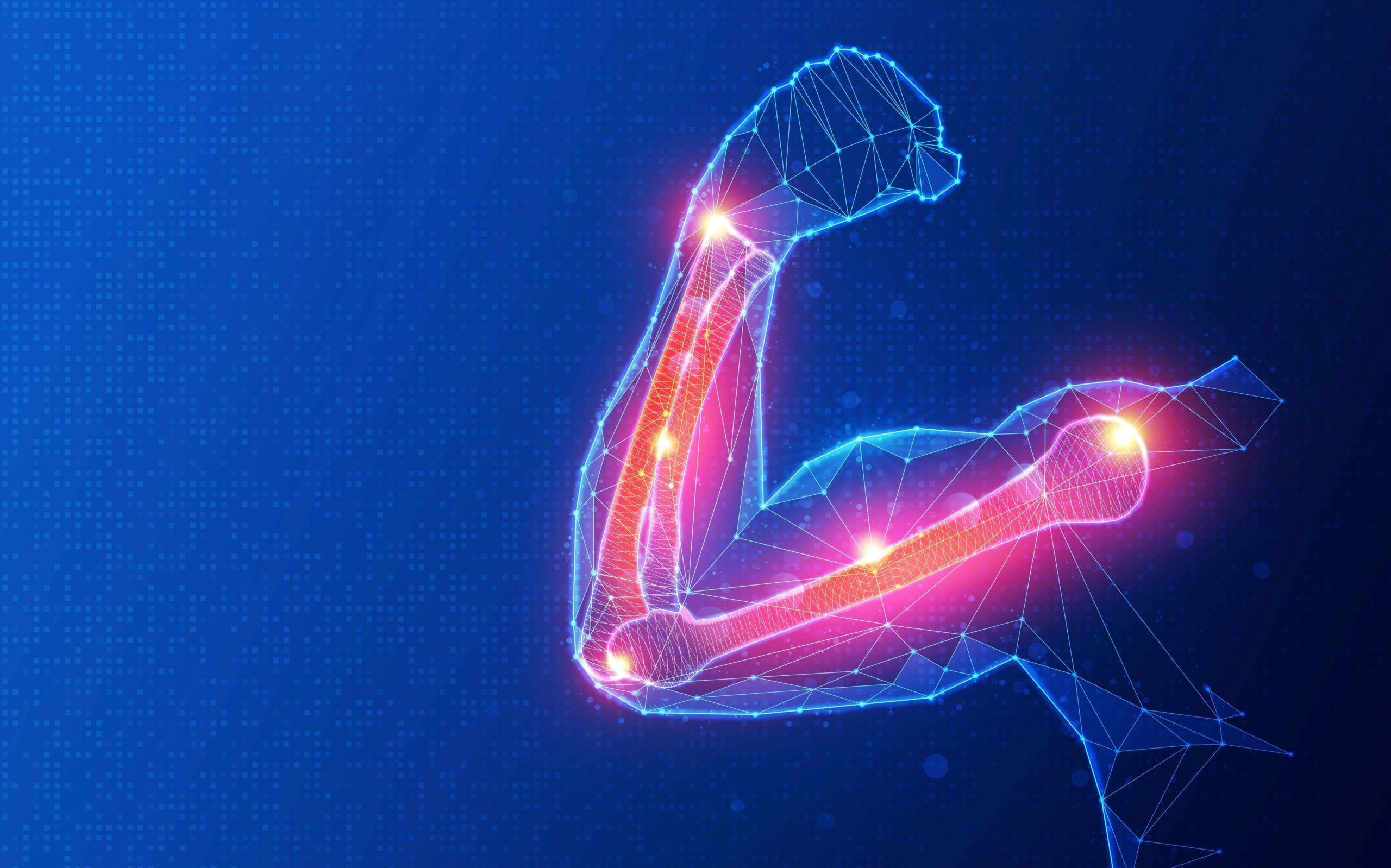
Electroactive biomaterials
Electrical signals play an important role in the healing and repair of various tissues. This phenomenon has contributed to the development of electroactive biomaterials that can produce electrical signals. Such biomaterials, together with tailored biophysical stimulation, can contribute significantly to the healing and regeneration of tissues such as bone, cartilage, nerves, muscles, and more. The use of electroactive biomaterials for the construction of sensors, transducers, actuators, generators, and more, which can be implanted or fused to the human body, offers broad prospects in disease treatment, diagnosis and health monitoring.
Piezoelectric biomaterials
Electrostimulation or bioelectricity in general is used in various therapies to treat musculoskeletal disorders, epithelial tissues, bone fractures and many others. However, the clinical use of electrostimulation therapies has its limitations due to the associated risks of increased spasticity, muscle pain, skin irritation and general inconvenience to patients. Therefore, piezoelectric materials are playing an increasingly important role because they accumulate charge and exhibit appropriate electrical activity in response to mild mechanical stresses (breathing, heartbeat, blood flow and gentle body movements, i.e. walking, running, etc.) that can be used for tissue regeneration.
In Greek, “piezo” means “induced by pressure,” meaning that piezoelectric materials can accumulate charge in the absence of an applied voltage and exhibit electrical activity in response to mild mechanical stresses. The materials undergo anisotropic changes that cause their dipole moment to exceed zero, providing piezoelectricity. Piezoelectric materials can generate low-level electric currents in tissues, mimicking the endogenous electric field to direct intracellular communication, ion transport and neuronal activity, profoundly affecting tissue regeneration. Piezoelectric biomaterials respond to even small physical stresses and can be actuated by vibration and ultrasound, making them a sustainable and self-powered approach in regenerative engineering. Examples of piezoelectric materials can be found among ceramics, polymers and composites, which are widely used in sensors, actuators, ultrasonic transducers and energy harvesting devices, although many of these materials exhibit various limitations.
Piezoceramic and piezopolymers
The most popular piezoceramic include bismuth sodium titanate (BNT), bismuth potassium titanate (BKT), sodium niobate, potassium niobate, potassium sodium niobate (KNN), and others. Although piezoceramic materials are very popular, their potential as biomaterial scaffolds is limited, mainly due to their non-biodegradable structure, structural rigidity, cytotoxicity and stiffness mismatch with natural tissues.
Therefore, piezoelectric polymers, also known as piezopolymers, are gaining popularity as they are lightweight and flexible, offer minimal mismatch in stiffness to tissue and biocompatibility, and are therefore more suitable for tissue regeneration applications. Piezopolymers are not without disadvantages, such as low piezoelectric constant, mechanical strength and durability, so piezocomposites combining the favorable features of these two groups of materials are becoming an increasingly interesting category of biomaterials to take advantage of the features of both categories of materials.
Examples of piezoelectric polymers refer mainly to poly(vinylidene fluoride)(PVDF), poly(L-lactide)(PLLA), polyamide, poly(3-hydroxybutyrate)(PHB) and collagen. These materials are most often processed into thin films or fibers. PVDF thin films exhibit piezoelectricity due to their ferroelectric chemical composition and have a piezoelectric coefficient ~6 pCN-1, which is about ten times higher than that of other polymers. PVDF has been used extensively in the development of piezobiomaterials, as tissue engineering is one such field dominated by PVDF. PLLA nanofibers produced by electrospinning exhibit also piezoelectric properties attributed to the presence of dipoles generated due to the presence of asymmetric carbon. An external electric field induced by shear deformation causes all PLLA atoms to move and change polarity. Overall, biodegradable piezoelectric materials are highly desirable for tissue regeneration, especially after implantation. Biodegradation is faster for piezoelectric polymers under physiological conditions compared to classical ceramics and composites.
Piezobiomaterials in tissue regeneration
Numerous examples of the use of electroactive biomaterials include the regeneration of soft tissues, such as skin or nerve tissue, and the regeneration of hard tissues, covering mainly bone and dentin. The delivery of low-level electrical currents through damaged tissue is a well-known phenomenon in nerve regeneration; however, the complexity and inconvenience of the electrical setup and external patient electrodes during administration have prompted the need for alternatives to electrical signaling. Given that nerve cells are electroactive, piezoelectric materials show great potential in regenerating the nervous system. In the presence of mechanical forces, piezoelectric materials can generate a piezopotential that activates voltage-gated ion channels to directionally transport electrical signals along axons for regeneration.
Piezopolymers have emerged as an excellent material that addresses the problems associated with current biomaterial-based nerve conduits by delivering appropriate electrical signals to nerve cells in the presence of a mechanical stimulus to promote nerve repair. To date, PVDF is the most widely used piezopolymer for nerve regeneration due to its high piezoelectricity, flexibility and excellent biocompatibility compared to other piezoelectric polymers.
Due to the piezoelectric nature of bone, piezoelectric signaling has also been found to modulate the metabolic activity of bone and promote osteogenesis. Therefore, developing materials with piezoelectric properties is a strongly explored area of bone tissue engineering as well. A wide range of studies have shown that piezoceramic materials can act as tissue activators and promote bone formation. One of the most studied piezoelectric ceramics is barium titanate oxide. The polar asymmetric structure induces piezoelectric behavior in BaTiO3, which occurs due to the displacement of Ti4+ and O2- ions. The biocompatibility of BaTiO3 enables bone regeneration in vitro by inducing osteoblast differentiation and hydroxyapatite deposition. It has also been found that PVDF has sufficient mechanical strength and acceptable biocompatibility for use in bone tissue engineering. The incorporation of PVDF into polycaprolactone (PCL) not only increased the piezoelectric coefficient of the composite, but also increased the tensile strength by 230% compared to the neat PCL scaffold.
Piezobiomaterials fabrication
While thin films and nanofibers exhibit the best piezoelectric properties, the past five years have seen the development of piezoelectric designs through additive manufacturing, including inkjet printing, 3D printing, etc., to achieve precise, multi-layered and customizable designs. 3D printing technology enables the fabrication of tissue scaffolds with complex designs, offering the ability to integrate sensors and other electrical components to develop wearable bioelectronics. This adaptability enables the production of biomimetic, flexible, wearable and implantable piezoelectric devices for tissue regeneration and real-time process monitoring. A strong impetus for accelerating the development of piezoelectric biomaterials is the involvement of artificial intelligence and deep learning algorithms to predict the output voltage by changing the size, composition and distribution of the biomaterial layer, which can be beneficial for monitoring cellular responses.
Summary
The development of piezoelectric biomaterials requires a critical look at various aspects. These include the selection of single or combinations of ceramic and polymer materials to achieve minimal tissue mismatch, electromechanical response, biocompatibility and flexibility for effective tissue regeneration. A deeper understanding of cell-material interactions to assess cell behavior, biocompatibility, tissue growth, elimination pathways and biodegradation of piezoelectric biomaterial is also crucial. In this context, density functional theory, molecular dynamics and other two-particle simulations can provide theoretical insights into biomaterial development and performance optimization. Considerable attention is required to study the surfaces and interactions between different materials in a piezocomposite to ensure that the range of piezoelectric power in response to ultrasound coincides with the endogenous electric field relevant to biomedical applications.
Author: Miroslawa El Fray
Link
[1] Amit Nain, Srishti Chakraborty, Snigdha Roy Barman, Pratik Gavit,
Sushma Indrakumar, Akhilesh Agrawal, Zong-Hong Lin, Kaushik Chatterjee, Progress in the development of piezoelectric biomaterials for tissue remodeling, Biomaterials, 2024, 307, 122528, https://doi.org/10.1016/j.biomaterials.2024.122528
Keywords
Piezoelectrics, Biomaterials, Ceramics, Polymers, Composites, Tissue remodeling