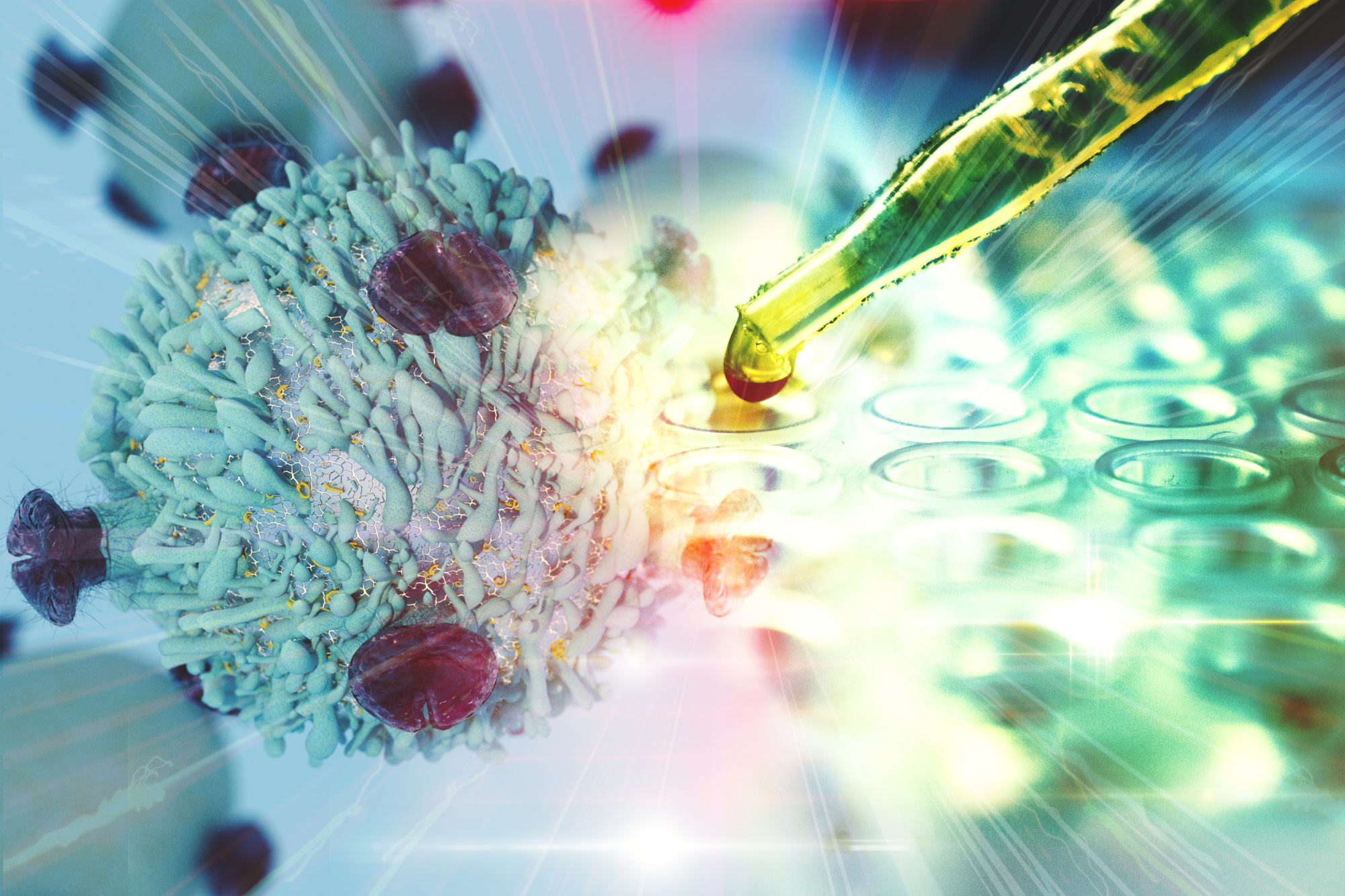
Biomaterials against Childhood Cancer
Introduction
The cellular ability to produce biomaterials may have major medical implications. This opens a range of possibilities, such as having a bank to manufacture a kidney, a liver or skin through the work of generating microtissues that cells create. At the moment, the different techniques that exist to generate and obtain these microtissues are diverse and have countless advantages and disadvantages.
Many studies focus on taking advantage of the interaction of stem cells such as those from the umbilical cord, adipose tissue, dental pulp, or bone marrow. Researchers have also worked with cartilage or skin.
The objective is to take medical advantage of the biomaterial that cells generate by themselves, characterising each of the cells and their behaviour under certain conditions to detail how the tissues they generate can be used.
Problem and current treatments
Advances in the treatment of haematological malignancies in paediatric and adult patients, based on the use of conventional treatments, such as hematopoietic stem cell transplantation and dose escalation of the different chemotherapies, have allowed the survival of these patients to improve over the last 20 years. However, survival has stabilized in recent years and a significant number of patients still relapse. Seeking improvements is difficult in treatments with chemotherapeutic agents because their intensification is limited by toxic side effects and is restricted by the plateau of efficacy. In addition, the spectrum of new drugs is depleting. For all these reasons, the need arises to develop new, more personalised and precise treatments that make it possible to break this current trend of survival stability, minimising its toxicity. In these new treatments we find the development of new therapeutic strategies based on targeted agents (precision medicine) and immunotherapy based on advanced therapy drugs (AT) and on the use of monoclonal antibodies against immune control points, among others.
Despite the fact that the survival of patients affected by haematological malignancies has improved in the last 20 years with chemotherapy and hematopoietic stem cell transplantation (HSCT), a significant number of patients still relapse. Some of the most significant advances have been related to HSCT, which led to a greater than 80% increase in the survival rate of acute lymphoblastic leukaemia (ALL) and a greater than 60% increase in leukaemia remission acute myeloid (AML) [1]. Allogeneic HSCT or donor lymphocyte infusions (DLI) [2] are already considered an immunotherapy strategy (although not AT, since it does not undergo a substantial manipulation phase), which in high-risk patients has decreased the risk of relapse due to its strong graft-versus-leukaemia (GVL) effects. On the other hand, the changes in the regimen, together with the escalation of doses, the combination of different chemotherapeutic drugs and the modifications in their formulations, have resulted in multiagent chemotherapy protocols, which have allowed better management of the disease and a better survival prognosis [3].
However, treatment intensification is limited by toxic side effects and restricted by plateauing efficacy, while the spectrum of new chemotherapeutic drugs is depleting. Therefore, the development of new therapeutic strategies based on targeted agents (precision medicine) and AT drug-based immunotherapy are currently in full swing. Developments in massive sequencing technologies [4,5], transcriptomic analysis [6,7], ultra-precise sequencing [8], optical genome mapping [9], and minimal residual disease detection [10, 11] have improved the accuracy of prognostic prediction and facilitated the use of targeted drugs against specific molecular targets [12]. On the other hand, the improvement in knowledge about cell cultures and genetic manipulation have allowed the development of immunotherapy strategies through advanced protocols of adoptive cell and gene therapies (adoptive cell therapy, ACT) [13].
Cancer immunosurveillance: the basis of immunotherapy
In recent decades, knowledge of the role of the immune system in antitumor surveillance has led to the development of novel therapeutic approaches that exploit the humoral and cellular branch of adaptive immunity, such as mAbs, cancer vaccines, and ACT. The ultimate goal of immunotherapy is to manipulate or activate the immune system so that it fights cancer. In addition, it is the goal of immunotherapy strategies to decrease toxicity against normal tissues by eliciting specific responses against tumour associated antigens (TAAs) and bypassing tumour immune escape/tolerance mechanisms [14].
There is a relationship between the immune system and cancer, so the development of new therapies based on the manipulation or use of components of the immune system (immunotherapies) have become a realistic therapeutic alternative in recent years. The tumour immune microenvironment (TIME) is part of the tumour microenvironment and is composed of subpopulations of lymphoid cells and myeloid cells that are involved in the cancer immunosurveillance process [15].
Immunosurveillance is the recognition and killing of cancer cells by lymphocytes, which act as sentinels recognising transformed cells with expression of tumour antigens that can activate various immune cell phenotypes [16]. It is critical to note that most of these antigens, except those derived from virus-infected cancer cells, are primarily self-proteins that are expressed with mutations or minor changes in their antigenic structure [17, 18]. During tumour progression, cancer cells show low immunogenicity, developing a series of strategies to inhibit the action of effector cells, so that these effector immune cells tolerate them, thus expanding and escaping immune control. The way in which cancer cells change in response to pressure from the immune system has been called immunological editing [15].
One of the mechanisms by which tumour cells evade the T-cell specific antitumor immune response is, for example, the loss of expression of MHC class I molecules, which leads to aberrant antigenic masking [18]. Furthermore, the MHC-peptide-T cell receptor complex elicited by a tumour antigen shows weak stability, since high affinity T cells tend to be tolerant to these antigens [16].
Another mechanism of direct inhibition induced by cancer cells is the expression on their surface of the ligand of two inhibitory molecules found on the surface of T cells: programmed cell death-1 (PD1) [19, 20] and cytotoxic T cell-associated protein (CTLA-4) [20, 21]. In this way, the inhibition of the activity of the lymphocytes is induced. Although these checkpoints often help modulate immune activation, they can also be used by tumour cells to inhibit the T cell antitumor response. PD1 is a transmembrane protein expressed on T, B, and NK cells and binds to ligands of PD1 (PD-L1 and PD-L2) in target cells. When bound to its ligand on tumour cells, PD1 inhibits tumour cell apoptosis, causes peripheral tumour cell apoptosis, causes depletion of peripheral effector T cells, and effector T cells on regulatory T cells. CTLA4 is also a physiological negative regulator of T cell activation. Interaction with CD80/CD86 on the tumour leads to inhibition of T cell function and suppression of their effector activity [21]. Knowledge of these two checkpoint inhibitors has opened the door to new antitumor therapeutic approaches. Furthermore, the interaction between tumour and non-tumour cells creates the tumour microenvironment, which for the most part is driven by tumour dynamics that promote the proliferation/expansion of cancer cells. For example, tumour and stromal cells release multiple factors, such as the chemokine CCL, which inhibit effector T cell functions and attract regulatory T cells (Tregs) into the microenvironment [16]. Tumour cells use different mechanisms for cancer progression and metastasis. Reactivating the antitumor effector response rate of the immune system, modulating some of the above points, is the objective of antitumor immunotherapy.
Types of immunotherapies
We can basically distinguish two types of immunotherapies:
- Passive immunotherapy
It consists of the transfer to tumour carriers of cells or antibodies previously generated in vitro and directed against the tumour [22]. The types of passive and specific immunotherapy are mAb and ACT: TIL and chimeric antigen receptor T (CAR-T) cells.
On the other hand, the use of LAK (lymphokine-activated killer) cells is a passive and non-specific immunotherapy, consisting of the culture of non-purified lymphocytes for 2-3 days with IL-2. Most of the cells that expand in this way are NK cells, so their antitumor effect is not restricted to MHC recognition.
- Active immunotherapy
Active immunotherapy intends to induce an immune response in vivo. Both passive and active immunotherapy can be directed specifically against tumour antigens (specific immunotherapy) or can aim at global activation of the immune system (non-specific immunotherapy).
Within active immunotherapy is the use of cytokines, included in non-specific immunotherapy, which allows activating the immune system in general; and vaccines, both for tumour antigens and for whole cells, which are included in specific immunotherapy strategies, because it is intended that the individual’s own immune system is being trained and activated against these antigens. The problem is that many of these antigens are either poorly known, as in the case of solid tumours, or poorly immunogenic, because the immune system has generated a tolerance to them.
Main immunotherapy strategies
Immunotherapy for hematologic malignancies encompasses cancer vaccines, cytokine administration, tumour-directed mAbs including immune checkpoint inhibitors (ICIs), drug-conjugated antibodies (ADC), oncolytic adenovirus, CAR-T cells, NK cells
Genetically modified chimeric antigen receptor (CAR-NK), bispecific T cell activating (BiTE) molecules, bispecific killer cell activator (BiKE), dual affinity retargeting (DART), and trispecific killer cell activating therapies (TriKE ) [23].
Immunotherapy based on advanced therapy drug developments
Advanced therapy drugs – definition
During the last decade, the traditional concept of “medication” has been expanded to incorporate new ATs. The development of ATs is complex, but they have incredible potential for the treatment of human diseases and are changing the paradigm in the treatment of numerous hereditary and acquired diseases, both of high and low prevalence, in which the use of conventional therapies is not enough.
Advanced therapy medicinal products are medicinal products for human use that are based on genes (gene therapy), cells (cell therapy) or tissues (tissue engineering), and include products of autologous, allogeneic, or xenogeneic origin. They constitute new therapeutic strategies, and their development will contribute to offering opportunities for some diseases that up to now lack effective treatments.
Drugs based on cell therapy: generalities and main indications
Cell therapy consists of the use of cells as therapeutic material to treat or prevent certain diseases. The main indications for cell therapy currently are tissue regeneration, the treatment of inflammatory or autoimmune diseases, mainly based on the immunoregulatory capacity of mesenchymal stem cells, and, finally, indications for cancer treatment, such as adoptive immunotherapy of T cells, specifically TILs.
Drugs based on gene therapy: generalities and main indications
Gene therapy consists of the transfer of genetic material to patient cells to treat or prevent pathologies, whether inherited or acquired, either by restoring, modifying, or enhancing cellular functions through the introduction of a functional gene into a target cell. Initially, gene therapy was restricted to patients with monogenic diseases. However, gene therapy is now also used for the treatment of more complex diseases. There are two approaches to performing gene therapy: ex vivo or in vivo treatment.
Depending on the strategy used to transfer the genetic material, gene therapy can be untargeted, where the genetic material is either not integrated (for example: oncolytic adenoviruses used as an antitumor strategy) or integrated into the cell genome, but without finding a specific site (integrative viral vectors, such as gamma-retrovirals and lentivirals).
Non-targeted gene therapy has already shown efficacy in patients with rare diseases with mutations in a variety of genes. These indications have been, for example, patients with severe combined immunodeficiencies, such as immunodeficiency caused by deficiency of the enzyme adenosine deaminase (SCID-ADA) or linked to the X chromosome (SCID-X1)., as well as other serious monogenic diseases, such as beta-thalassemia, sickle cell anaemia or Fanconi anaemia.
Within cancer gene therapy, strategies using oncolytic virotherapy have shown efficacy for solid tumours with few therapeutic options. A Spanish group has developed a combined therapy using cell therapy and oncolytic virotherapy for extracranial and brain tumours in children and adults with uveal melanoma. Another Spanish group, that of Dr. Marta Alonso, has recently published the encouraging result of a clinical trial for the treatment with an oncolytic virus of the paediatric tumour with a very poor prognosis DIPG (diffuse intrinsic pontine glioma).
When gene therapy is targeted, the integration of the exogenous sequence is directed to a specific location in the genome. This last modality is known as gene editing. It consists of the use of enzymes called nucleases, such as the CRISPR/Cas system, which specifically recognize DNA sequences and “cut” the DNA molecule, generating a double-strand break (DSB). In the presence of a template DNA, it can be repaired by homologous recombination and introduce a specific sequence into the genome, making it possible, for example, to correct mutations in a gene. In the absence of template DNA, the DSB is repaired by non-homologous end ligation, which will introduce small insertions or deletions that cause, in most cases, the interruption of the gene, as is the case of the PD-1 gene, which is a negative regulator of lymphocyte functionality; thus, PD-1 gene modification is being developed to optimise immunotherapy to treat cancer.
The first EMA-approved AT drug, Strimvelis®, is composed of CD34+ cells transduced with a retroviral vector for the treatment of ADA-SCID. Two ex vivo T cell gene therapy drugs have recently been approved for the treatment of neoplasms, tisagenlecleucel and axicabtagene ciloleucel, based on redirecting antigenicity recognition of T cells by a chimeric sequence between the sScFv sequence of an antibody and activation domains of the T cell receptor, TCR. They are called CAR (chimeric antigen receptor) and, the final product, CAR-T cells.
Drugs based on tissue engineering: generalities and main indications
A tissue engineered product is defined by the AEMPS as one that contains or is made up of cells or tissues manipulated by engineering and which is alleged to have properties, is used or administered to people to regenerate, restore or replace human tissue. An example is artificial skin generated from a skin biopsy from which fibroblasts and keratinocytes are isolated and expanded. After a period of expansion, both cell types are assembled to generate skin implants to be placed in the patient.
This technology is used for the recovery of burned skin. This technology is also being used as a therapeutic strategy in combination with gene therapy in genetic skin diseases, such as epidermolysis bullosa, which is caused by mutations in the COL7A1 gene.
Conclusion
This article has explained the definition of the most important concepts related to immunotherapy: what it is, what its action is based on, what technological developments have allowed and what types of immunotherapies are there, as they are being carried out in the paediatric Hospital Niño Jesús in Madrid.
These concepts have been described, as well as the specific strategies included in each group of immunotherapies, stopping at the most widely used therapeutic strategies which are being used in the clinic, and that, due to technological advances related to massive sequencing, the development of gene transfer technology and advances in cell culture techniques, they are having a great expansion due to their therapeutic efficacy.
References
[1]. Wayne AS, Reaman GH, Helman LJ. Progress in the curative treatment of childhood hematologic malignancies. J Natl Cancer Inst. 2008;100(18):1271-3.
[2]. Weiden PL, Sullivan KM, Flournoy N, Storb R, Thomas ED. Antileukemic effect of chronic graft-versus-host disease: contribution to improved survival after allogeneic marrow transplantation. N Engl J Med. 1981;304(25):1529-33.
[3]. Lee-Sherick AB, Linger RMA, Gore L, Keating AK, Graham DK. Targeting paediatric acute lymphoblastic leukaemia: novel therapies currently in development. Br J Haematol. 2010;151(4):295-311.
[4]. Bannon SA, Routbort MJ, Montalban-Bravo G, Mehta RS, Jelloul FZ, Takahashi K, et al. Next-Generation Sequencing of DDX41 in Myeloid Neoplasms Leads to Increased Detection of Germline Alterations. Front Oncol. 2021;10:582213.
[5]. Montalbán-Bravo G, Kanagal-Shamanna R, Sasaki K, Patel K, Ganan-Gómez I, Jabbour E, et al. NPM1 mutations define a specific subgroup of MDS and MDS/MPN patients with favorable outcomes with intensive chemotherapy. Blood Adv. 2019;3(6):922-33. https://ashpublications.org/bloodadvances/article/3/6/922/246728/NPM1-mutations-define-a-specific-subgroup-of-MDS.
[6]. Chien KS, Class CA, Montalbán-Bravo G, Wei Y, Sasaki K, Naqvi K, et al. LILRB4 expression in chronic myelomonocytic leukemia and myelodysplastic syndrome based on response to hypomethylating agents. Leuk Lynphoma. 2020;61(6):1493-9. https://www.tandfonline.com/doi/abs/10.1080/10428194.2020.1723014.
[7]. Sakhdari A, Class C, Montalbán-Bravo G, Sasaki K, Bueso-Ramos CE, Patel KP, et al. Immunohistochemical loss of enhancer of Zeste Homolog 2 (EZH2) protein expression correlates with EZH2 alterations and portends a worse outcome in myelodysplastic syndromes. Modern Pathology. 2022;35(9):1212-9. http://www.modernpathology.org/article/S0893395222002769/fulltext.
[8]. Short NJ, Kantarjian H, Kanagal-Shamanna R, Sasaki K, Ravandi F, Cortes J, et al. Ultra accurate Duplex Sequencing for the assessment of pretreatment ABL1 kinase domain mutations in Ph+ ALL. Blood Cancer J. 2020;10(5):61. https://www.nature.com/articles/s41408-020-0329-y.
[9]. Yang H, García-Manero G, Sasaki K, Montalbán-Bravo G, Tang Z, Wei Y, et al. High-resolution structural variant profiling of myelodysplastic syndromes by optical genome mapping uncovers cryptic aberrations of prognostic and therapeutic significance. Leukemia. 2022;36(9):2306-16: https://www.nature.com/articles/s41375-022-01652-8.
[10]. Jabbour E, Short NJ, Jorgensen JL, Yilmaz M, Ravandi F, Wang SA, et al. Differential impact of minimal residual disease negativity according to the salvage status in patients with relapsed/refractory B-cell acute lymphoblastic leukemia. Cancer. 2017;123(2):294-302. https://onlinelibrary.wiley.com/doi/full/10.1002/cncr.30264.
[11]. Short NJ, Kantarjian HM, Sasaki K, Cortes JE, Ravandi F, Thomas DA, et al. Prognostic significance of day 14 bone marrow evaluation in adults with Philadelphia chromosome–negative acute lymphoblastic leukemia. Cancer. 2016;122(24):3812-20. https://onlinelibrary.wiley.com/doi/full/10.1002/cncr.30262
[12]. Sasaki K, Ravandi F, Kadia T, DiNardo C, Borthakur G, Short N, et al. Prediction of survival with intensive chemotherapy in acute myeloid leukemia. Am J Hematol. 2022;97(7):865-76. https://onlinelibrary.wiley.com/doi/full/10.1002/ajh.26557
[13]. Nogami A, Sasaki K. Therapeutic Advances in Immunotherapies for Hematological Malignancies. Int J Mol Sci. 2022;23(19):11526.
[14]. Dougan M, Dranoff G. Immune therapy for cancer. Annu Rev Immunol. 2009;27:83-117.
[15]. Kim R, Emi M, Tanabe K. Cancer immunoediting from immune surveillance to immune escape. Immunology. 2007;121(1):1-14.
[16]. Sukari A, Nagasaka M, Al-Hadidi A, Lum LG. Cancer Immunology and Immunotherapy. Anticancer Res. 2016;36(11):5593-606.
[17]. Galon J, Bruni D. Tumor Immunology and Tumor Evolution: Intertwined Histories. Immunity. 2020;52(1):55-81.
[18]. Garrido F, Cabrera T, Concha A, Glew S, Ruiz-Cabello F, Stern PL. Natural history of HLA expression during tumour development. Immunol Today. 1993;14(10):491-9.
[19]. Seidel JA, Otsuka A, Kabashima K. Anti-PD-1 and Anti-CTLA-4 Therapies in Cancer: Mechanisms of Action, Efficacy, and Limitations. Front Oncol. 2018;8:86.
[20]. Lee HT, Lee SH, Heo YS. Molecular Interactions of Antibody Drugs Targeting PD-1, PD-L1, and CTLA-4 in Immuno-Oncology. Molecules. 2019;24(6):1190.
[21]. Bengsch F, Knoblock DM, Liu A, McAllister F, Beatty GL. CTLA-4/CD80 pathway regulates T cell infiltration into pancreatic cancer. Cancer Immunol Immunother. 2017;66(12):1609-17.
[22}. Weber EW, Maus M V, Mackall CL. The Emerging Landscape of Immune Cell Therapies. Cell [Internet]. 2020;181(1):46-62.
[23}. Varadé J, Magadán S, González-Fernández Á. Human immunology and immunotherapy: main achievements and challenges. Cell Mol Immunol. 2021;18(4):805-28.
Keywords
Cancer, immunotherapies, cell culture, gene therapy, cell therapy