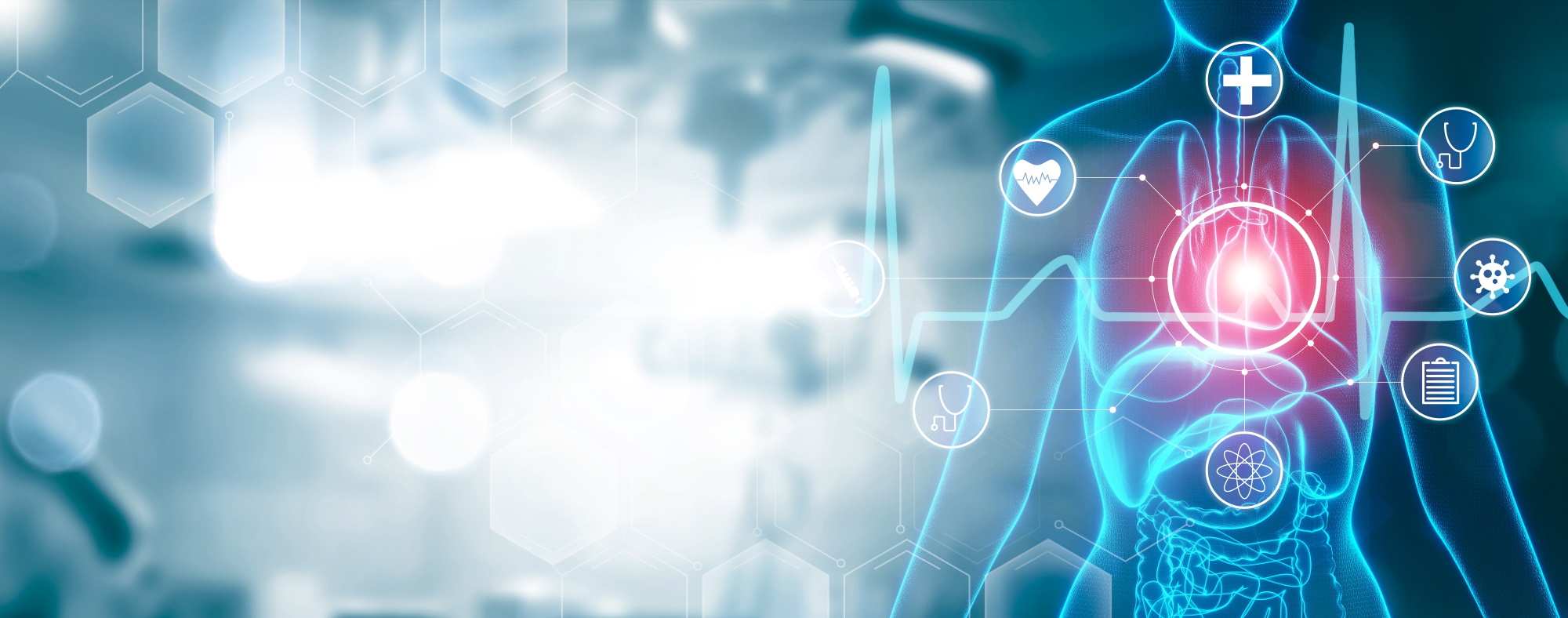
Biomaterials and medical devices for organ treatments
The great and steady increase of research in biomaterials and medical devices over the past decades has led to novel opportunities for treatments of organs. Different biomaterials and medical devices can be used for different body parts and treatment methods, such as soft-tissue repair, oral and dental devices, and more. The following article provides a detailed overview of various biomaterials and medical devices and their use for the treatment of organs.
Organ Systems Commonly Treated with Biomaterials/Medical Devices
In recent years, many biomaterials have been used in combination with medical devices either alone or in combination (Table 1). Single or individual materials with well-established properties, such as metal alloys for structural support or bone and joint replacement, such as titanium and its alloys, allow for their common use. The most flexible polymers are used in multipurpose catheters or lubricants made from silicone.
Table 1 Examples of Biomaterial Types and Uses. Table modified from [1], Table 11.1, p 431–2.
Biomaterial | Characteristics and advantages | Disadvantages | Examples |
Metals and alloys | Strong, ductile (some metals are better than others) | Corrosion, dense, hard to manufacture |
|
Stainless steel (316L) | Alloy of iron, nickel, chromium, manganese | Fatigue failure, corrosion, nickel release | Plates, screws, rods for fractures, endovascular stents |
Titanium and titanium-based alloys (Ti-Al-V, Ti-Al-Nb) | Osseointegration | Not high strength | Bone and joint replacement (stem portion that is inserted into the marrow cavity) |
Cobalt-based alloys (Co-Cr, Co-Cr-Mo) |
| Wear debris in joints (nano) | Joint replacements (Hard joint surfaces) |
Tantalum (porous) | Flexible, corrosion resistant, | Pure tantalum is heavy, soft metal; difficult to machine | Orthopedic implants |
Nickel titanium (Nitinol) | Shape memory alloy: nickel not released from titanium | Difficult to manufacture | Stents, endovascular, gynecologic use |
Gold | Malleable, inert | Expensive | Dental restoration, pacemaker; nanoparticles |
Platinum | Ductile | Scarce metal | Electrodes |
Ceramics and glasses |
| Brittle |
|
Alumina, zirconia | Wear resistant compared with metal-on-metal or metal-on-polymer surfaces | Brittle | Joints |
Calcium phosphates (Tricalcium phosphate, calcium hydroxyapatite, bicalcium phosphates) | osteoconduction, osseointegration | Calcium phosphate cements: poor mechanical strength, slow degradation | Bone substitutes, bone repair, potential for drug delivery |
Bioactive glasses |
| Stiff, brittle | Bone repair, bone substitute |
Polymers | Resilient | Not as strong as other materials, deforms over time, may degrade |
|
Polyethylene |
| Wear debris (large) | Articulating joint surfaces |
Polypropylene, polyamides | Nonabsorbable |
| Suture materials |
Silicone | Easy to form, durable, hydrophobic |
| Soft tissue implants, tubing, anticoagulant coating for syringes and needles |
Polymethyl methacrylate (PMMA) |
|
| Bone cement |
Hydrogels (natural or synthetic), hyaluronic acid | Hydrophilic, biodegradable |
| Delivery of drugs or biologics, tissue scaffolding |
Composites | Combined advantages of components | Complex, potentially longer regulatory approval |
|
Metal-polymer | Drug delivery to reduce or slow onset of sequelae |
| Drug-eluting stents, heart valve replacement |
Ceramic-resorbable polymers (collagen, polysaccharides) | Improved porosity |
| Bone substitute |
Extra-corporal bioartificial organ devices: fiber or porous matrix or scaffolding with functioning cells | Bioreactor restoration of function | Technically complex, expensive to design and maintain | Extracorporeal liver support (investigational) |
Metal-ceramic | Hard surface of joints prevent wear; HA coatings improve osseointegration |
| Joint surfaces; calcium apatite coating with delivery of biomolecules |
Natural or biologics |
|
|
|
Collagen (polymer) |
|
| Stents |
Glutaraldehyde cross-linked porcine valves or bovine pericardium |
| Xenograft, calcification leading to failure | Heart valve replacement |
Abbreviations: HA, hydroxyapatite; PMMA, polymethyl methacrylate.
Cardiovascular
Owing to the unique blood contact challenges and hemodynamic conditions of cardiovascular devices, the most common failure is caused by structural failure, such as fatigue rupture of the leaflets for heart replacement valves, degradation, calcification, and thrombosis.
Heart replacement valves have historically been performed using mechanical valves, usually made from a composite of polymers and metals. Bioprosthetic valves that encompass xenografts, such as porcine valves or bovine pericardium, homografts, such as valves from live or dead human donors, and autografts, such as valves from another part of the body of the same patient, have emerged as alternatives to heart valve replacement. Nonclinical evaluation of cardiac devices has recently been reviewed. [2, 3]
Musculoskeletal
Humans have been using different types of musculoskeletal implants with the aim of repairing bones, joints, and tendon injuries. For more than a century, implants of bone obtained from another part of the body of the same patient (autogenous), from another human (allogeneic), and from another species (xenogenic) have been used to repair bone defects. Different sources of bone grafts have different risks including viral transmission.
Some bone substitutes are intended to replace the full bone;, some to replace part of the bone, and others are intended as bone void fillers with no structural support. Different objectives require different implant criteria for each application, such as long bone fracture repair, periodontal augmentation, or arthroplasty procedures. The sum of these issues, along with the necessity for materials with different properties that can be used in various applications, have motivated extensive and intensive investigations and development on this topic. The microscopic evaluation of orthopedic devices has recently been reviewed [4].
Ophthalmic Devices
The ocular system comprises a compartmentalised complex architecture. This conformation confers several possibilities for placement of ophthalmic devices. The possible application routes of non-drug delivery devices include injection or implantation (Table 2). Various ocular devices, biomaterials, and drug-delivery devices have been extensively reviewed. [5, 6]
The combination products of a biomaterial and a drug, small molecule, or biologic, are drug delivery devices. These devices are designed to gradually release the drug in specific places or ocular compartment. These intraocular inserts, especially those designed for intra-vitreous implantation, represent a promising field of application because they allow access to intraocular compartments that are hardly accessible via the vascular route for controlled drug release.
The ISO 10993-6:2016 standard establishes a preference for small laboratory animal models, such as mice, rats, hamsters, and rabbits. In practice, rabbits are the most commonly used animals for testing. Large animals, such as minipigs, dogs, and human primates, are used less frequently for biocompatibility and performance studies of ophthalmic devices.
Table 2 Routes of Application of Ocular Medical Devices Table modified from [1], Table 11.6, p 459.
Application route | Examples | Comments |
Intravitreal, posterior segment injection or implantation | Endo-tamponade agents for temporary (gas) or permanent (silicone oil) replacement of the vitreous | Action relying on physical and/or rheological properties (no pharmacologic activity) |
Lens capsular bags, intraocular implantation | Intraocular lens (IOLs) | Implanted within the lens capsular bag following the removal of cataractous lens fibres by phacoemulsification |
Intracorneal implantation | Keratoprostheses | Inserted in the corneal stroma to replace diseased cornea following graft failure |
Scleral implantation | Scleral buckles | Polymer implants pinned through the sclera to treat retinal detachment |
Intraorbital implantation | Ocular prosthesis (“glass eye”) | Used for total eye replacement procedure, ceramic or bioactive glass prosthesis |
Subretinal/epiretinal implantation | Retinal prosthesis | Nitinol-based prostheses implanted either in the suprachoroidal space or the subretinal space, or lying on the retina (epiretinal) |
Subconjunctival or suprachoroidal implantation | Shunts | Plastic or metallic glaucoma- drainage devices implanted in the suprachoroidal or subconjunctival spaces to drain excessive aqueous humor |
Anterior segment injection | Ophthalmic viscosurgical devices | To protect the corneal endothelium during anterior segment surgery |
Topical | Contact lenses, eye drops, and gels | Drops and gels, no pharmacologic activity in the eye
|
Soft Tissue Repair and Wound
Suture materials and associated grids, sponges, and meshwork implants are extensively employed as biomaterials and implanted devices during surgical interventions. These devices play a pivotal role in soft tissue repair procedures. Sutures and similar materials can be broadly categorised into two types based on their properties: absorbable and non-absorbable.
Absorbable sutures are specifically engineered to undergo degradation over a certain period. Examples of absorbable sutures include chromic gut sutures, polyglycolic acid, polylactic acid, polydioxanone, and caprolactone. In contrast, nonabsorbable materials consist of diverse substances, such as silk, metals (e.g., stainless steel sutures and staples), synthetic plastics (e.g., nylon sutures), and meshes (e.g., polypropylene, polyester, and PTFE).
Recently, there has been a comprehensive examination of the histological characteristics of meshes, as discussed in the review conducted by Keating et al. in 2019 [7]. Evaluation of these biomaterials and implanted devices is crucial for understanding their tissue interactions, biocompatibility, and long-term performance. Such assessments will contribute to improving surgical techniques and enhancing patient outcomes in soft tissue repair.
Neural and Endocrine Devices
The range of diseases and conditions targeted by devices interacting with or physically altering the central (CNS ¼ brain and spinal cord) and peripheral (PNS ¼ ganglia and nerves) nervous systems is rapidly expanding. These conditions encompass various ailments, such as movement disorders, pain modulation, and genetic storage diseases. Neural devices available for clinical use can be classified as active or passive.
Active neural devices deliver electrical or thermal energy to exert their therapeutic effects. Examples include neural stimulators, which are implanted devices capable of providing deep electrical signals to specific regions of the brain (used for Parkinson’s disease), spinal cord (for severe chronic back pain), vagal nerve (for epilepsy and depression), sacral nerve (for urinary bladder control in cases of spinal cord injury), and cochlea (for certain types of deafness). On the other hand, passive neural devices encompass drug delivery devices and shunts.
Cerebral shunts, commonly employed in the treatment of hydrocephalus, are implanted into one of the brain ventricles to facilitate the drainage of excess fluid into the peritoneal cavity. Temporary or chronic intrathecal catheters, introduced into a brain ventricle or the epidural space, are frequently used to deliver biomolecules directly to tissues within the CNS.
These neural devices offer promising therapeutic interventions for various neurological conditions. The development and advancement of such devices improve the quality of life for patients affected by these disorders by providing targeted and effective treatment options.
Oral/Dental
Oral and dental devices that aim at replacement and restoration encompass a range of applications. These devices are designed to replace soft tissue, such as gingival grafts, and teeth, which involve abutments for dental implants, root canal amalgam, metals, cements, resins, and other materials. Additionally, there are devices specifically created to prevent soft tissue ingrowth, such as membranes, and devices focused on enhancing oral bone structure, including bone void fillers and other articles discussed previously in the musculoskeletal section. [8, 9]
In certain cases, multiple devices may need to undergo simultaneous testing. For example, a bone void filler test article may be evaluated in conjunction with a marketed membrane to assess its effectiveness in preventing soft tissue ingrowth following surgery.
The development and evaluation of these oral and dental devices play a vital role in advancing dental restoration and replacement procedures. Rigorous testing and assessment ensure their safety, efficacy, and long-term performance, ultimately contributing to improving outcomes.
Bioengineered Implants, Combination Devices, and Delivery Systems Combination Devices
Devices can be enhanced by incorporating bioactive substances, such as serving as a delivery system or providing a scaffold for cells. This integration allows for applications like drug depots, gene therapy delivery, and tissue engineering. The resulting composites can closely resemble autografts by combining synthetic scaffolding with host cells, where tissue pieces are transferred within the same individual. Depending on the desired outcome, devices can facilitate rapid release of bioactive materials, as seen in catheters. Alternatively, they can be designed to enable delayed or sustained release by complexing the bioactive component, such as antibiotics bound to hydroxyapatite for implantation into contaminated sites within injured bones.
Other Organ Systems and Devices
The field of medical devices encompasses a wide range of instruments, equipment, and supplies used in various medical applications. These include surgical instruments, imaging devices, diagnostic equipment, patient care and monitoring equipment, as well as hospital supplies. Generally, non-implanted devices as well as Class I and some Class II devices for any organ system do not typically require nonclinical evaluations.
Toxicity studies with histopathologic evaluation are commonly conducted on medium-risk (Class II) and high-risk (Class III) implanted medical devices. However, even low-risk medical devices that are new to the market or involve a specific tissue may necessitate toxicity studies with comprehensive histopathologic assessments until comparable reference devices, known as predicates, become available.
Toxicologic pathologists primarily evaluate medical devices intended for cardiovascular, ophthalmic, orthopaedic, wound management, neurologic, and dental indications. Providing pathology support for medical devices falling outside these major market indications, devices utilising novel biomaterials or exhibiting unique activity, and devices associated with infrequently evaluated tissues or tissue compartments can pose particular challenges.
The evaluation of medical devices in organs and tissues that require infrequent assessment includes the urogenital, respiratory, and gastrointestinal systems, as well as body cavities and the ear. In many cases, evaluations of devices in these tissues can be adapted from assessments conducted on predicate devices in other tissues and body cavities, such as stents, catheters, meshes, embolic beads, gels, and laser applications.
However, when it comes to implants in the ear, challenges arise due to the unique anatomy, histopathology, and interpretation difficulties even for experienced pathologists familiar with other medical devices. In recent years, medical devices and combination products have grown increasingly complex in structure. They have been further complicated by the presence of subcutaneous implanted electronic modules and cutaneous sensors, the use of tunable biodegradable materials, the development of novel absorbable metallic implants, the introduction of self-forming gels and thermoresponsive materials, and the incorporation of immunomodulatory surface modifications or combination products that release immunomodulatory molecules. [10-13]
Note: This article is based on Wancket, L.M., J.C. Schuh, and E. Drevon-Gaillot, Biomedical materials and devices, in Haschek and Rousseaux’s Handbook of Toxicologic Pathology, Volume 2: Safety Assessment Environmental Toxicologic Pathology. 2023, Elsevier
Author: Juan Uribe-Gomez, Bohara Raghvendra
References
[1] Wancket, L.M., J.C. Schuh, and E. Drevon-Gaillot, Biomedical materials and devices, in Haschek and Rousseaux’s Handbook of Toxicologic Pathology, Volume 2: Safety Assessment Environmental Toxicologic Pathology. 2023, Elsevier. p. 427-466.
[2] Leigh Perkins, L., Preclinical models of restenosis and their application in the evaluation of drug-eluting stent systems. Veterinary pathology, 2010. 47(1): p. 58-76.
[3] Perkins, L.E.L. and M.K. Rippy, Balloons and stents and scaffolds: preclinical evaluation of interventional devices for occlusive arterial disease. Toxicologic Pathology, 2019. 47(3): p. 297-310.
[4] Jackson, N., et al., Histopathological evaluation of orthopedic medical devices: the state-of-the-art in animal models, imaging, and histomorphometry techniques. Toxicologic Pathology, 2019. 47(3): p. 280-296.
[5] Lloyd, A.W., R.G. Faragher, and S.P. Denyer, Ocular biomaterials and implants. Biomaterials, 2001. 22(8): p. 769-785.
[6] Nagaraj, R., et al., A review on recent advancements in ophthalmology devices: Currently in market and under clinical trials. Journal of Drug Delivery Science and Technology, 2019. 52: p. 334-345.
[7] Keating, J.H., R. Melidone, and F. Garcia-Polite, Preclinical evaluation of mesh implants: The pathologist’s perspective. Toxicologic Pathology, 2019. 47(3): p. 379-389.
[8] Pearce, A., et al., Animal models for implant biomaterial research in bone: a review. Eur Cell Mater, 2007. 13(1): p. 1-10.
[9] Gibbs, S., et al., Biology of soft tissue repair: Gingival epithelium in wound healing and attachment to the tooth and abutment surface. Eur. Cells Mater, 2019. 38: p. 63-78.
[10] Chagnon, M., L.-G. Guy, and N. Jackson, Evaluation of magnesium-based medical devices in preclinical studies: challenges and points to consider. Toxicologic Pathology, 2019. 47(3): p. 390-400.
[11] Rousselle, S.D., et al., Pathology of bioabsorbable implants in preclinical studies. Toxicologic Pathology, 2019. 47(3): p. 358-378.
[12] Rousselle, S.D., et al., Histology strategies for medical implants and interventional device studies. Toxicologic Pathology, 2019. 47(3): p. 235-249.
[13] Yogev, S., et al., Local toxicity of topically administrated thermoresponsive systems: in vitro studies with in vivo correlation. Toxicologic Pathology, 2019. 47(3): p. 426-432.
Keywords
biomaterials, medical devices, organs, treatments, implants, tissue repair, organ systems, wounds, dental